Honey Bee Navigation, Learning and Memory
A Bee Has to Learn Color and Shape of Flowers
Clarence Collison
“Foraging is essential to a honey bee colony’s survival. To forage successfully, a bee has to learn and remember not only the color and shape of flowers that contain nectar and pollen, but also how to get to them (Menzel et al. 1996; Collett et al. 2003). Since the species of flowers that are in bloom, say, in the morning are likely to be replaced by a different species at a different location in the afternoon, the bee needs, and has indeed evolved, an impressive ability to learn and memorize local features and routes, as well as the time of blooming, quickly and accurately. Thus, having found a nectar bearing flower at a particular time on a particular day, a forager can remember the task and the time at which it was completed, and visit the flower at the same place and time on the following day (von Frisch 1967; Lindauer 1960) (Zhang et al. 2006).”
“Honey bees use visual attributes of the targeted food source, such as its color, shape, size, direction and distance from the hive, and the landmarks around it to navigate during foraging. They transmit the location information of the food source to other bees if it is highly rewarding. To investigate the relative importance of these attributes, Kheradmand et al. (2020) trained bees to feeders in two different experiments. In the first experiment, they asked whether bees prefer to land on (a) a similar feeder at a different distance on the same heading or on (b) a visually distinct feeder located at the exact same location. They found that, within a short foraging range, bees relied heavily on the color and the shape of the food source and to a lesser extent on its distance from the hive. In the second experiment, they asked if moving the main landmark or the feeder (visual target) influenced recruitment dancing for the feeder. They found that foragers took longer to land and danced fewer circuits when the location of the food source, or a major landmark associated with it, changed. These results demonstrate that prominent visual attributes of food sources and landmarks are evidently more reliable than distance information and that foraging bees heavily utilize these visual cues at the later stages of their journey.”
“Honey bees were trained to fly from their hive to a feeding site along a flight path marked by visually discriminable landmarks 3.46 meters high. The landmarks were placed at regular intervals of 90 meters. During training, the feeder was located directly in front of the third (target) landmark. In the tests, bees were given the choice of landing at the trained distance or at the target landmark which had been displaced to a different distance. Bees preferred to land at the target landmark when the discrepancy between the two indicated distances was small, but landed mostly at the trained distance if the discrepancy was large. Furthermore, distance estimation depended on landmarks encountered during flight. When the target landmark was placed at a distance from the hive closer than the trained distance, the bees’ readiness to respond to this landmark could be increased only if bees had already passed the landmark that preceded the target landmark during training. Thus, a given flight goal is defined in the bees’ memory by its distance from the hive, landmarks that mark the goal, and landmarks that precede the goal during flight. At the same time, the bees, as a group, exhibited considerable flexibility to land in places that were defined by only one or two of the cues (Chittka et al. 1995).”
“In both their navigation and dance communication, bees are able to compensate for the sun’s movement. When foragers are prevented from seeing the sun for two hours, they compensate by extrapolation, using the sun’s rate of movement when last observed. These and other data suggest a time-averaging processing strategy in honey bee orientation (Gould 1980).”
“Foraging bees embark on their feeding flights and return to the hive using sun compass information (Wehner and Menzel 1990), and visual distance estimation (Esch and Burns 1996; Srinivasan et al. 1996; Menzel et al. 1996). These sources of information are tightly connected: compass directions are derived from extended landmarks (e.g. when bees fly along the edge of a forest) and home vectors are associated with local landmarks (Menzel et al. 1998), establishing a memory for the flight route between the hive and feeding site. It thus appears that spatial navigation in bees is not a unitary process but involves multiple navigational systems (Menzel et al. 2000).”
“Honey bees navigate using disparate (essentially different in kind; not allowing comparison) visual cues at different parts of their route. Upon exiting the hive, the bee has access to limited stimuli: landmarks around the hive and direction information from the e-vector. She can use these cues to travel in the correct direction. On her route, she can compare the real-time visual input of near and far-range landmarks to memories of points on the route on her previous trips and maintain her heading while keeping track of how far she has travelled. Once near her floral destination, she can use short-range landmarks to pinpoint her target (Collett et al. 2013). These navigational strategies broadly fall into three categories: alignment imaging matching, positional image matching, and path integration (Collett et al. 2013). At each part of the path, if the necessary visual information is available, bees can use it to navigate. Alignment image matching provides a simple way of comparing current views or cues extracted from a view with memories from previous trips. The animal then tries to maximize the match through corrective movements. If the bee is not too far from known areas where familiar features are abundant, this strategy allows her to stay on course. Positional image matching works by extrapolating the current location from differences between relationships among parts of the current view image and the same relationships in memories of views. The only requirement for positional image matching is that the panoramas (landscapes) of the novel area and the known areas share sufficient items in common. Alignment and positional image matching are not necessarily achieved in different ways, rather they are different scaling’s of using memorized visual information to orient towards a goal. Path integration does not require landmarks, but only a source of the directional reference point (offered by the sun and its e-vector) and a source odometry (optic flow or stride counting). By keeping track of the path segments taken, the animal can ideally integrate its current location at any moment and find a homing vector (Collett et al. 2013). The relative accuracy of these three strategies depends on the situation. Path integration has a relatively low error at shorter distances and in novel areas but can result in larger errors when orienting near the goal because the remaining vector is much smaller than the accumulated error. Alignment image matching requires familiarity with the route but can guide the animal with minimal error and can be learned in a single trip. Landmarks clearly play key roles in alignment and positional image matching strategies but can also be combined with path integration to create short vectors for segments of the entire route (von Frisch 1967) (Kheradmand and Nieh 2019).”
“Free-flying bees were conditioned on a vertical wall to a vertical tactile pattern consisting of parallel lines of grooves and elevations. The asymptote of the learning curve is reached after approximately 25 rewards. Bees can discriminate the conditioned vertical pattern from a horizontal or diagonal alternative. Angle discrimination is apparent only for relatively coarse tactile cues. The proboscis extension response of fixed bees was used to condition bees to a vertical tactile pattern which was presented to the antennae. The learning curve reaches an asymptote after four rewards. After seven unrewarded extinction trials the conditioned responses are reduced to 50%. Bees show best discrimination for patterns whose edges they can scan with their antennae. The animals show a high degree of generalization by responding to an object irrespective of the trained pattern. Under laboratory conditions fixed bees can discriminate the angles and spatial wavelengths of fine tactile patterns consisting of parallel grooves. Bees can also discriminate forms and sizes of tactile patterns. They do not discriminate between different types of edges and between positive and negative forms (Erber et al. 1998).”
“Searching for reward motivates and drives foraging behavior. In honey bees, specialized pollen foragers are attracted to and learn odors with pollen. However, the role of pollen as a reward remains poorly understood. Unlike nectar, pollen is not ingested during collection. Nery et al. (2020) hypothesized that pollen (but not nectar) foragers could learn pollen by sole antennal or tarsal stimulation. Then, they tested how pairing of pollen (either hand- or bee collected) and a neutral odor during a pre-conditioning affects performance of both pollen and nectar foragers during the classical conditioning of the proboscis extension response. Secondly, they tested whether nectar and pollen foragers perceive the simultaneous presentation of pollen (on the tarsi) and sugar (on the antennae) as a better reinforcement than sucrose alone. Finally, they searched for differences in learning of the pollen and nectar foragers when they were prevented ingesting the reward during the conditioning. Differences in pollen-reinforced learning correlate with division of labor between pollen and nectar foragers. Results show that pollen foragers performed better than nectar foragers during the conditioning phase after being preconditioned with pollen. Pollen foragers also performed better than nectar foragers in both the acquisition and extinction phases of the conditioning, when reinforced with the dual reward. Consistently, pollen foragers showed improved abilities to learn cues reinforced without sugar ingestion.”
“The honey bee is a model organism for studies on the neural substrates of learning and memory. Associative olfactory learning using sucrose rewards is fast and reliable in foragers and older hive bees. However, researchers have so far failed to show any significant learning in newly emerged bees. It is generally argued that in these bees only part of the brain structures important for learning are fully developed. Here, Behrends and Scheiner (2009) show for the first time that newly emerged bees are capable of associative learning, if they are sufficiently responsive to sucrose. Responsiveness to sucrose, which can be measured using the proboscis extension response (PER), increases with age. Newly emerged bees are on average very unresponsive to sucrose. They showed that if newly emerged bees displaying a PER to 10% sucrose or lower sucrose concentrations are conditioned to an odor, they show significant associative learning and early long-term memory. Nevertheless, the level of acquisition is still lower than in foragers. The general assumption that newly emerged bees are incapable of associative learning must therefore be reconsidered. Their study suggests that an age-dependent increase in responsiveness to rewarding stimuli is directly related to the development of early learning abilities.”
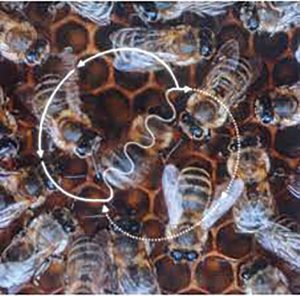
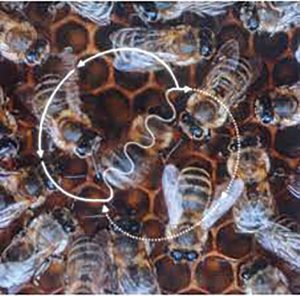
“The cognitive abilities of queens are not well understood, although queen learning and memory are essential when virgin queens leave the hive to go on their mating flight(s) and successfully navigate back to the hive. Honey bee queen learning has never been previously demonstrated. Gong et al. (2018) tested olfactory learning in queens and workers and examined the role of DNA methylation, which plays a key role in long-term memory formation. They provided the first evidence that honey bee queens have excellent learning and memory. The proportion of honey bee queens that exhibited learning was five-fold higher than that of workers at every tested age and, for memory, 4-fold higher than that of workers at a very young age. DNA methylation may play a key role in this queen memory because queens exhibiting remote memory had a more consistent elevation in Dnmt3 gene expression as compared with workers. Both castes also showed excellent remote memory (seven day memory), which was reduced by 14-20% by the DNA methylation inhibitor zebularine. Given that queens live approximately 10-fold longer than workers, these results suggest that queens can serve as an excellent long-term reservoir of colony memory.”
“Honey bee drones do not forage for themselves and/ or for their colony, however, they require learning to navigate toward a specific location associated with their mating behavior; drone congregation areas and their home hive. They normally return to their colony during their mating flights to refuel if they were unsuccessful in mating with a queen. Hayashi and Satch (2021) tested whether homing in drones results from learning the landscape around their hives.
They compared the homing success between drones released at sites that were familiar to them (resident group) and those released at unfamiliar sites (transported group). Drones from the resident group were able to return to their hive, whereas those from the transported group could not, with the exception of a few drones that were released close to the sites that offered direct visual information for the drones to return to the hives. These results indicate that drones learn about their hives and the surrounding landscape during their flights.”
References
Behrends, A. and R. Scheiner 2009. Evidence for associative learning in newly emerged honey bees (Apis mellifera). Anim. Cog. 12: 249-255.
Chittka, L., K. Geiger and J. Kunze 1995. The influences of landmarks on distance estimation of honey bees. Anim. Behav. 50: 23-31.
Collett, M., L. Chittka and T.S. Collett 2013. Spatial memory in insect navigation. Curr. Biol. 23: R789-R800.
Collett, T.S., P. Graham and V. Durier 2003. Route learning by insects. Curr. Opin. Neurobiol. 13: 718-725.
Erber, J., S. Kierzek, E. Sander and K. Grandy 1998. Tactile learning in the honeybee. J. Comp. Physiol. A 183: 737-744.
Esch, H.E. and J.E. Burns 1996. Distance estimation by foraging honeybees. J. Exp. Biol. 199: 155-162.
Gong, Z., K. Tan and J.C. Nieh 2018. First demonstration of olfactory learning and long-term memory in honey bee queens. J. Exp. Biol. 221: 1-10.
Gould, J.L. 1980. Sun compensation by bees. Science 207: 545-547.
Hayashi, S. and T. Satch 2021. Landscape learning during flight ensures homing in honey bee (Apis mellifera) drones. J. Insect Behav. 34: 82-88.
Kheradmand, B. and J.C. Nieh 2019. The role of landscapes and landmarks in bee navigation: a review. Insects 10: 342.
Kheradmand, B., J. Cassano, S. Gray and J.C. Nieh 2020. Influence of visual targets and landmarks on honey bee foraging and waggle dancing. Insect Sci. 27: 349-360.
Lindauer, M. 1960. Time-compensated sun orientation in bees. Cold Spring Harb. Symp. Quant. Biol. 25: 371-377.
Menzel, R., K. Geiger, L. Chittka, J. Joerges, J. Kunze and U. Müller 1996. The knowledge base of bee navigation. J. Exp. Biol. 199: 141-146.
Menzel, R., K. Geiger, J. Joerges, U. Müller and L. Chittka 1998. Bees travel novel homeward routes by integrating separately acquired vector memories. Anim. Behav. 55: 139-152.
Menzel, R., R, Brandt, A. Gumbert, B. Komischke and J. Kunze 2000; Two spatial memories for honeybee navigation. Proc. R. Soc. Lond. B. 267: 961-968.
Nery, D., E, Moreno and A. Arenas 2020. Pollen reinforces learning in honey bee pollen foragers but not in nectar foragers. J. Exp. Biol. 223: 1-11.
Srinivasan, M.V., S.W. Zhang, M. Lehrer and T.S. Collett 1996. Honeybee navigation en route to the goal: visual flight control and odometry. J. Exp. Biol. 199: 237-244.
von Frisch, K. 1967. The Dance Language And Orientation Of Bees, Harvard University Press,
Cambridge, MA., 566 pp.
Wehner, R. and R. Menzel 1990. Do insects have cognitive maps? Ann. Rev. Neurosci. 13: 403-414.
Zhang, S., S. Schwarz, M. Pahl, H. Zhu and J. Tautz 2006. Honeybee memory: a honeybee knows what to do and when. J. Exp. Biol. 209: 4420-4428.
Clarence Collison is an Emeritus Professor of Entomology and Department Head Emeritus of Entomology and Plant Pathology at Mississippi State University, Mississippi State, MS.